Prof. QIAO Zhenhua from Hefei National Laboratory for Physical Sciences at Microscale and Department of Physics and collaborators achieved new breakthroughs on quantum anomalous Hall effect in graphene and silicene systems. The results are respectively published in
Phys. Rev. Lett. 112, 106802 (2014) and
Phys. Rev. Lett. 112, 116404 (2014). The latter one has been selected as “Editors’ Suggestions”.
Quantum anomalous Hall effect is one of the most attractive research topics in the current Condensed Matter Physics field. The conventional quantum Hall effect arises from the Landau-level quantization in the presence of a strong magnetic field. As a new quantum state of matter, quantum anomalous Hall effect originates from the joint effect of spin-orbit coupling and the local exchange field. This effect was theoretically proposed by an American scientist F. D. M. Haldane in 1988. After numerous explorations in various new quantum materials, it is finally realized in a magnetically doped topological insulator by an international research group conducted by Prof. XUE Qikun from Tsinghua University at an ultra-low temperature [Science 340, 167 (2013)]. It is highly valuable from both theoretical and practical sides to study the quantum anomalous Hall effect at a higher temperature or in some other easily realizable materials.
Because of its peculiar crystal structure and linear Dirac energy dispersion [See panels (a) and (c)], graphene provides another ideal platform for exploring quantum anomalous Hall effect. Different from topological insulators, graphene itself is nonmagnetic and has an extremely weak intrinsic spin-orbit coupling. In 2010, Dr. QIAO Zhenhua and collaborators proposed that by introducing the mirror-symmetry breaking Rashba spin-orbit coupling and time-reversal symmetry breaking exchange field, graphene can open a topologically nontrivial bulk band gap to host the quantum anomalous Hall effect [Phys. Rev. B 82, 161414(R)(2010)]. In the subsequent projects, their team has done a series of studies to reveal the microscopic mechanism of its formation and provided several experimental prototypes, for example, adsorbing periodically or randomly distributed magnetic metal atoms in graphene. Unfortunately, the adatoms in graphene tend to form clusters rather to form diluted distribution, indicating that it is extremely difficult to realize quantum anomalous Hall effect in graphene via adsorbing magnetic atoms.
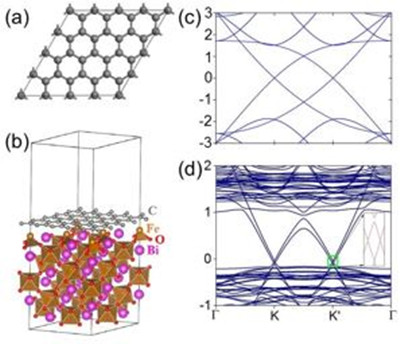
Fig. 1: (a) The 4x4 supercell of graphene; (b) The 4x4supercell of graphene on top of the (111) ferromagnetic plane of BiFeO3; (c) Band structure corresponding to panel (a) [gapless Dirac bands]; (d) Band structure corresponding to panel (b) [gapped Dirac bands].
Prof. QIAO Zhenhua and collaborators proposed a new experimental scheme to realize the quantum anomalous Hall effect: by placing graphene on top of the magnetic plane of an antiferromagnetic insulator (e.g. BiFeO3) [see panel (b)]. Due to the proximity effect between graphene and the magnetic atoms, a relatively larger extrinsic Rashba spin-orbit coupling and a very strong exchange field are induced to open a quantum anomalous Hall band gap corresponding to the temperature of around 11.5 K [see panel (d)]. In addition, by applying a vertical stress to decrease the distance between graphene and the magnetic substrate, the proximity effect can be enhanced to increase the experimentally realizable temperature up to 40 K [See details in Phys. Rev. Lett. 112, 116404 (2014)].
As a cousin material of graphene, silicene is composed of silicon atoms with honeycomb structures. In addition to the exceptional properties of graphene, the ripple structure of silicene gives rise to a larger intrinsic spin-orbit coupling and intrinsic Rashba spin-orbit coupling than those in graphene. Due to its strong intrinsic spin-orbit coupling, silicene is regarded as an ideal material to realize the quantum spin-Hall effect. When the time-reversal symmetry is broken, the intrinsic Rashba spin-orbit coupling can also lead to the quantum anomalous Hall effect. Prof. Zhenhua Qiao and collaborators theoretically found that the quantum anomalous Hall effect from either intrinsic or extrinsic Rashba spin-orbit coupling has the same contribution at different valley points in the momentum space. However, whenever the intrinsic and extrinsic Rashba spin-orbit couplings are simultaneously present, its joint effect produces a valley-polarized quantum anomalous Hall effect, i.e. the contributions from different valleys are different to the quantum anomalous Hall effect, which makes this new state of matter exhibit the characteristics of both quantum anomalous Hall effect and quantum valley Hall effect. This work provides a solid theoretical basis for designing low-power valleytronics [See details in Phys. Rev. Lett. 112, 106404 (2014)].
The research was financial supported by USTC, Bairen Program of CAS, NNSFC, and Synergetic Innovation Center of Quantum Information and Quantum Physics.
(School of Physical Sciences, Hefei National Laboratory for Physical Sciences at Microscale)