Recently, a research team led by Prof. LI Chuanfeng from the University of Science and Technology of China (USTC) achieved a breakthrough in quantum photonics. They developed an on-chip photonic simulator capable of simulating arbitrary-range coupled frequency lattices with gauge potential. This study was published in Physical Review Letters.
The quest for effective simulators that can replicate the dynamics of real systems has been a driving force in quantum physics. Photonic systems, with their ability to control properties like polarization and frequency, have emerged as versatile candidates for quantum simulation. However, the challenge lies in creating frequency lattices that can simulate complex structures like atom chains and nanotubes, which are crucial for understanding low-dimensional materials.
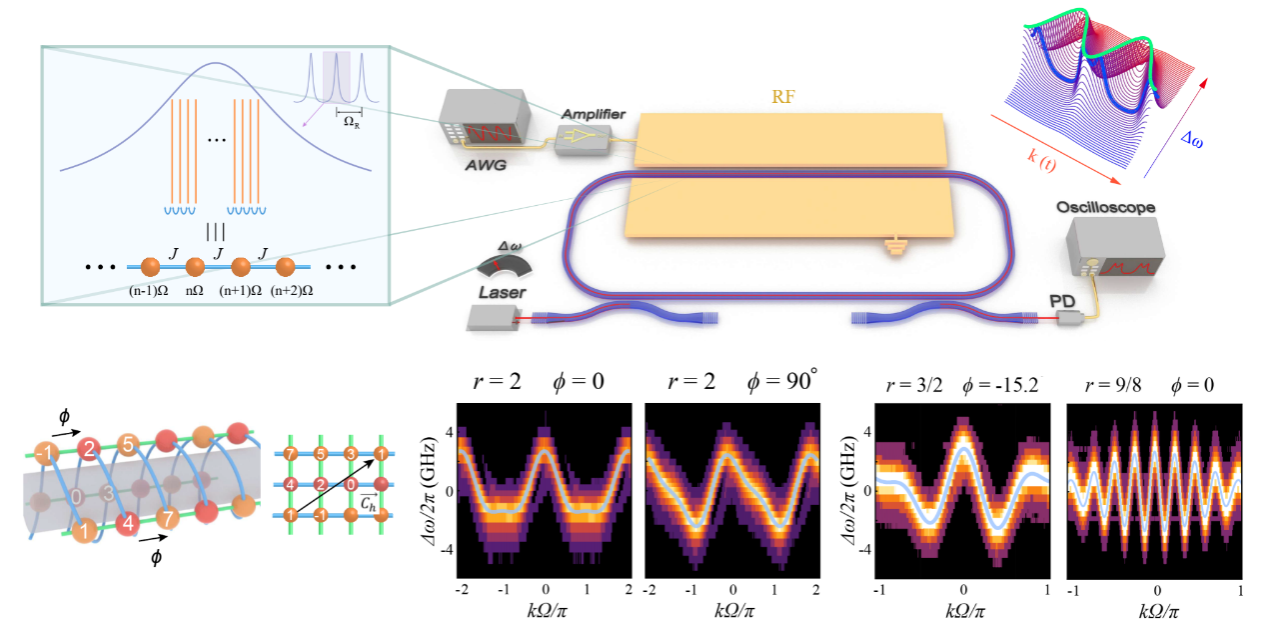
Scenario and the experimental setup. (Image from USTC)
To address this challenge, the team's innovative approach involves the use of thin-film lithium niobate chips, which are particularly suited for creating lattices in the frequency domain due to their high electro-optic coefficient. By periodically modulating an on-chip resonator, the researchers observed band structures, a significant advancement as it allows for the simulation of structures with arbitrary-range coupling. Remarkably, their method enabled coupling up to 8 and 9 times the lattice constant while reducing the required modulation frequency by over five orders of magnitude. This is achieved by including multiple lattice points within one resonant peak, which alleviates the difficulty of applying and detecting multiharmonic signals conventionally of ultrahigh frequency on chips.
In this study, the special focus on low-frequency radio-frequency modulation offers a high degree of flexibility in choosing lattice points and regulating compound interaction. This approach significantly reduces the required frequencies by more than three orders of magnitude, translating to a reduction from near 100 GHz to around 10 MHz in their examples. This not only simplifies the design and fabrication challenges but also lessens the demands on source and measurement equipment.
This work not only greatly alleviates the difficulties posed by high frequencies in on-chip synthetic dimensions but also maintains the scalability of traditional implementation methods, allowing it to be extended to higher-dimensional models. It achieves high-dimensional and complex frequency synthetic dimensions on thin-film lithium niobate optical chips. The reviewers highly praised the achievement, stating it "opens a new avenue within the area of studying synthetic dimensions on photonic chips."
Paper link: https://doi.org/10.1103/PhysRevLett.133.233805
(Written by GE Shuyun, edited by WU Yuyang, USTC News Center)